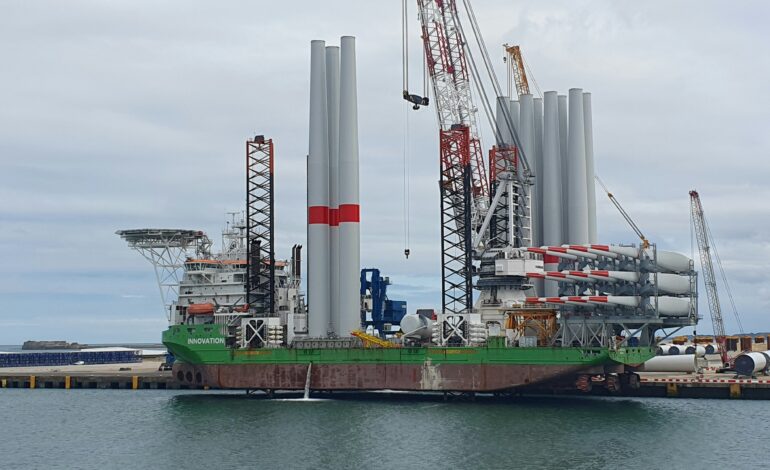
Carbon Footprint Analysis of Fossil Fuels vs. Alternative Marine Fuels
Introduction
The shipping industry is a significant contributor to global greenhouse gas (GHG) emissions, primarily due to the extensive use of bunker fuels. As concerns about climate change intensify, the maritime sector faces increasing pressure to reduce its carbon footprint. Alternative marine fuels, such as liquefied natural gas (LNG), hydrogen, ammonia, and biofuels, are emerging as potential solutions to this challenge. This article provides an in-depth carbon footprint analysis of traditional bunker fuels compared to these alternative marine fuels, examining their environmental impacts, technological feasibility, and future prospects.
Understanding Carbon Footprint in Marine Fuels
The carbon footprint of marine fuels refers to the total amount of GHG emissions produced throughout the fuel’s lifecycle, including extraction, production, transportation, and combustion. Key metrics include carbon dioxide (CO2), methane (CH4), and nitrous oxide (N2O) emissions, expressed in CO2-equivalent (CO2e) terms.
Bunker Fuels: Heavy Fuel Oil (HFO) and Marine Gas Oil (MGO)
Lifecycle Emissions
- Extraction and Production: The extraction and refining of crude oil into HFO and MGO are energy-intensive processes, resulting in substantial upstream emissions.
- Transportation: Transporting bunker fuels to ports and storage facilities adds to their carbon footprint.
- Combustion: Burning HFO and MGO in ship engines produces significant CO2, sulfur oxides (SOx), nitrogen oxides (NOx), and particulate matter (PM).
Environmental Impact
- High CO2 Emissions: HFO and MGO have high carbon content, leading to significant CO2 emissions per unit of energy produced.
- Air Pollution: The combustion of HFO, in particular, releases high levels of SOx and NOx, contributing to air pollution and acid rain.
Regulatory Pressure
- IMO Regulations: The International Maritime Organization (IMO) has implemented regulations such as the IMO 2020 sulfur cap, limiting sulfur content in marine fuels to 0.5%. This has led to a shift towards MGO and low-sulfur fuel oils (LSFO).
- Carbon Intensity Targets: IMO aims to reduce the carbon intensity of international shipping by at least 40% by 2030 and 70% by 2050, compared to 2008 levels.
Alternative Marine Fuels
Liquefied Natural Gas (LNG)
- Lifecycle Emissions: LNG offers a lower carbon footprint compared to traditional bunker fuels. It produces about 20-25% less CO2 during combustion. However, methane slip during production and transportation can offset some of these benefits.
- Technological Feasibility: LNG infrastructure is expanding, with increasing numbers of LNG-powered ships and bunkering facilities. However, the high cost of retrofitting existing vessels remains a barrier.
- Environmental Impact: LNG significantly reduces SOx and NOx emissions, contributing to improved air quality.
Hydrogen
- Lifecycle Emissions: Hydrogen can be produced via electrolysis using renewable energy (green hydrogen) or from natural gas (grey or blue hydrogen). Green hydrogen offers a near-zero carbon footprint, while grey and blue hydrogen have higher emissions.
- Technological Feasibility: Hydrogen fuel cells and combustion engines are under development, but widespread adoption requires significant advancements in storage, handling, and safety technologies.
- Environmental Impact: Hydrogen combustion produces only water vapor, eliminating CO2, SOx, and NOx emissions.
Ammonia
- Lifecycle Emissions: Ammonia can be produced from renewable electricity or fossil fuels. Green ammonia, produced using renewable energy, has a low carbon footprint, while traditional methods result in higher emissions.
- Technological Feasibility: Ammonia can be used in internal combustion engines and fuel cells, but it poses challenges related to toxicity, storage, and handling.
- Environmental Impact: Ammonia combustion emits no CO2, but it can produce NOx, which requires control technologies to mitigate.
Biofuels
- Lifecycle Emissions: Biofuels are derived from organic materials and can offer significant carbon savings, particularly if produced sustainably. Lifecycle emissions depend on feedstock, production methods, and land-use changes.
- Technological Feasibility: Biofuels can be blended with traditional marine fuels or used as drop-in replacements, making them a flexible option for existing ship engines.
- Environmental Impact: Biofuels generally produce lower SOx and NOx emissions compared to bunker fuels, but their sustainability depends on feedstock sourcing and production practices.
Comparative Analysis
Carbon Intensity
- HFO and MGO: Highest carbon intensity due to high carbon content and inefficient combustion.
- LNG: Lower carbon intensity, but methane slip can be a significant issue.
- Hydrogen (Green): Near-zero carbon footprint, highly sustainable.
- Ammonia (Green): Low carbon footprint, but NOx emissions need management.
- Biofuels: Variable carbon intensity, generally lower than traditional fuels, but dependent on sustainable production practices.
Cost and Infrastructure
- HFO and MGO: Established infrastructure, but facing increasing regulatory and environmental costs.
- LNG: Growing infrastructure, high initial investment for retrofitting ships.
- Hydrogen: Requires significant investment in new infrastructure and safety measures.
- Ammonia: Similar to hydrogen, with additional challenges in handling toxicity.
- Biofuels: Compatible with existing infrastructure, but supply chain and scalability are concerns.
Regulatory and Policy Considerations
- HFO and MGO: Increasingly stringent regulations driving a shift to low-sulfur alternatives and cleaner fuels.
- LNG: Supported by policies promoting lower emissions, but methane slip is a regulatory focus.
- Hydrogen and Ammonia: Future-oriented policies aimed at decarbonization are encouraging research and development.
- Biofuels: Incentivized by policies supporting renewable energy and sustainable practices, but sustainability criteria are critical.
Conclusion
The transition from traditional bunker fuels to alternative marine fuels is essential for reducing the carbon footprint of the maritime industry. While HFO and MGO are currently the dominant fuels, their high carbon intensity and environmental impact necessitate a shift towards cleaner alternatives. LNG, hydrogen, ammonia, and biofuels each offer distinct advantages and challenges.
LNG presents a practical interim solution with lower emissions but requires careful management of methane slip. Hydrogen and ammonia offer substantial long-term benefits with near-zero carbon footprints, but their adoption hinges on technological advancements and infrastructure development. Biofuels provide a flexible and immediate option for reducing emissions, though their sustainability depends on responsible sourcing and production.
Ultimately, achieving significant reductions in the maritime industry’s carbon footprint will require a combination of these alternative fuels, supported by robust policies, technological innovation, and industry collaboration. As the industry moves towards a more sustainable future, the integration of these alternative fuels will play a pivotal role in meeting global climate goals and ensuring the long-term viability of maritime transport.